The forest industry produces a range of products including paper, lumber, and energy, wielding substantial economic influence in numerous nations. Its significance extends to contributing significantly to each country’s GDP, generating substantial revenue, and offering employment to a large workforce, often in rural areas. Beyond economic benefits, forests also play a vital role in providing ecological advantages, encompassing carbon sequestration and biodiversity conservation.
The forest supply chain comprises integrated value chains such as pulp and paper mills, lumber production units, biorefineries, and energy plants. Its uniqueness lies in the involvement of multiple public and private organizations—ranging from small to large-scale companies, governments, Indigenous communities, hunters, and tourist representatives—each influenced by socioeconomic, technological, and environmental forces. Despite common goals, conflicting objectives among these entities often surface. Unlike manufacturing industries that reduce product numbers in the production process, forest value chains diverge, increasing product variety along the chain, thereby presenting unique planning challenges.
Complexity and challenges
Planners typically adopt a “push” approach due to the complexities arising from divergent flows. Advanced planning tools based on optimization for divergent industries are scarce, leading to ad hoc coordination among stakeholders, commonly established through contractual terms that engage only one partner at a time. The diverse data requirements for value chains involve extensive resources and processes, often characterized by high uncertainty in estimating natural resource volumes and qualities. Moreover, transportation activities—via trucks, trains, and vessels—span vast geographical areas.
Strategic, tactical, and operational planning in various fields align with long-term, medium-term, and short-term perspectives. For instance, strategic planning in forest transportation might encompass a five-year span, perhaps to invest in a terminal or new machine system. Such a timeframe, although strategic in transportation, would be considered tactical in forest management. The strategic forest management model, which can extend over 100 years in certain forest regions like boreal forests, encapsulates numerous variables that might not directly translate to immediate business decisions but rather anticipate their potential impact. Despite models projecting over 100 years, actual business decisions are implemented over significantly shorter periods, usually a few years. In the realm of planning models, categorization often entails classifying them as strategic if decisions span over a year or more, tactical for models covering one to twelve months, and operational for decisions made within shorter periods, sometimes less than a month. Additionally, there are real-time decision models addressing business choices made within minutes. The scientific literature displays a broad and sometimes overlapping use of these planning horizon terminologies.
Operations Research (OR) in forestry has evolved intertwined over the past 50 years, significantly influenced by methodological advancements tailored to specific forestry issues. For instance, the 1970s introduced Linear Programming (LP) solvers for forest management or network flow problems. Geographic Information Systems (GIS) and remote sensing technologies revolutionized forest management optimization models, incorporating spatial data for tasks like harvest scheduling and climate-aware management. These advancements also supported transportation and other value chain activities. Below we illustrate a few application areas that frequently appears in forest planning.
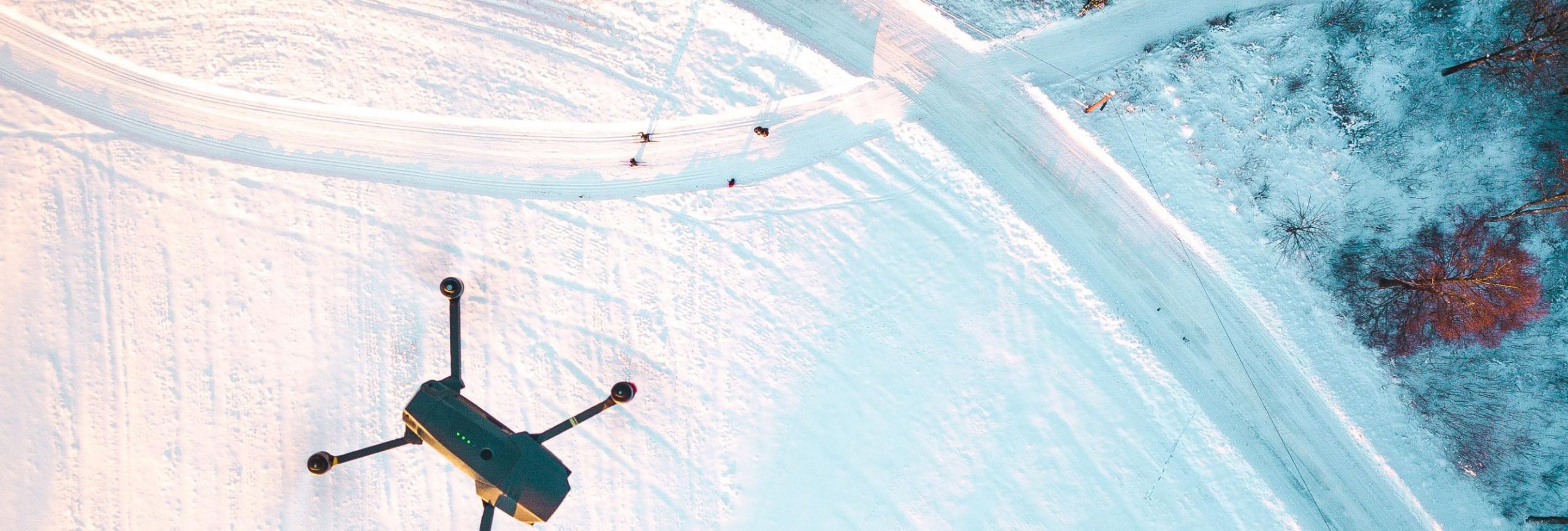
Strategic forest management models delve into the intricate interplay between management decisions—like harvest scheduling—and critical concerns such as sustainability, biodiversity preservation, ecosystem health, soil and water quality, social impacts, and economic returns. These models are geared towards making long-term decisions, often spanning the cycle between planting and harvesting. Such strategic planning occurs at both industry and government levels. While fire is a noticeable natural disturbance process impacting forest management, other factors like insects, diseases, and weather—though less dramatic in appearance—equally affect harvest operations, transportation logistics, and the overall value of forests.
Forest road design remains a central network problem in forest infrastructure, often integrating transportation and harvesting decisions to anticipate road construction needs. Network design models, typically formulated as Mixed Integer Programming (MIP) models, cover multiple time periods, spanning several years, and are vital for facilitating harvesting and access to harvest areas.
Harvest planning, a key facet, involves selecting specific areas for cutting during a planning period while considering the demand for various assortments. Each harvest area comprises different assortments with specific volumes. Decision-making about harvesting involves a mix of 0/1 variables for logical decisions and continuous variables for production and transportation, typically leading to large Mixed Integer Programming (MIP) models. In the 1990s, solving such models was constrained by size, prompting the proposal and utilization of heuristic methods. With the development of efficient solvers, it is now possible to solve large sized problems. Growing environmental concerns, including visual disturbance, habitat fragmentation, and social impacts of harvesting, led to constraints limiting the size of adjacent harvest areas. Other environmental considerations emphasized the need for large continuous patches of old-growth forests for unhindered wildlife movement. More short term operational harvest planning includes assigning specific harvest teams to the areas and also decide about the sequencing while taking into account demand at industriues.
Bucking, the process of dividing felled trees into logs, depends on various factors like tree species, log diameter, and quality, determining log length and the final product. This process can occur at the harvest area, carried out manually by chainsaw operators or through mechanized harvesters. The latter use on-board bucking optimization software to establish the best bucking given a price list for the different log types. The resulting logs are collected, transported to landings, and further moved to terminals or mills using forwarder machines or other methods. Advanced measurement systems are sometimes employed for optimized bucking and considering specific demands.
Transportation activities involve moving products—such as roundwood, trees, forest residue, and wood chips—between supply and demand points, utilizing trucks, vessels, or trains. Planning approaches range from aggregated flows to detailed operational routing, incorporating specifics like truck capacity and costs. Forest routing, though aligned with the Vehicle Routing Problem (VRP) class, encompasses unique characteristics defined by pickup and delivery points, time periods, inventory considerations, and network structures reflecting supply, demand points, and possible transportation roads.
To read more: Reference [1] provides a general description of how Operations research has contributed to forestry. Reference [2] describes the general forest value chain, and [3] focusses more on collaboration and strategic value chain planning. Reference [4] discuss 33 open problems in forestry. Ref [5-8] provides some typical applications where optimization has been a vital tool in developing decision support systems or as a support in analysis and development.
[1] M. Rönnqvist, D. Martell, A. Weintraub, Fifty Years of Operational Research in Forestry, International Transactions in Operational Research, Vol. 30, 3296-3328, 2023.
[2] The Management of Industrial Forest Plantations, Eds J.G. Borges, L. Diaz-Balteiro, M.E. McDill and L.C.E. Rodriguez, Springer, 2014
[3] Forest Value Chain Optimization and Sustainability, Eds S. D’Amours, M. Ouhimmou, J.-F. Audy and Y. Feng, CRC Press, 2016
[4] M. Rönnqvist, S. D’Amours, A. Weintraub, A. Jofre, E. Gunn, R.G. Haight, D. Martell, A.T. Murray, C. Romero, Operational Research challenges in forestry: 33 Open Problems, Annals of Operations Research, Vol. 232, 11-40, 2015.
[5] P. Flisberg, M. Frisk, M. Rönnqvist, Integrated harvest and logistic planning including road upgrading, Scandinavian Journal of Forest Research, Vol. 29, Suppl. 1, 195-209, 2014.
[6] P. Flisberg, M. Frisk and M. Rönnqvist, FuelOpt : A decision support system for forest fuel logistics, Journal of the Operational Research Society, Vol. 63, 1600-1612, 2012.
[7] D. Carlsson, S. D’Amours, A. Martel and M. Rönnqvist, Supply chain management in the pulp and paper industry, INFOR, Vol. 47(3), 167-183, 2009.
[8] H. Broman, M. Frisk and M. Rönnqvist, Supply chain planning of harvest operations and transportation after the storm Gudrun, INFOR, Vol. 47(3), 235-245, 2009.